Show Transcript
The chip is roughly half the size of a credit card, but the action takes place in a stamp-sized glass enclosure set in the center. Through the glass, the unaided eye can detect narrow rows of parallel lines, which resolve, under magnification, into channels lined with thousands of miniscule pens. When the chip is in use, thousands of living cells flow into the channels, and single cells are encased inside boxes of light and gently escorted into the pens. The chip and its enabling technologies operate as a nanoscale laboratory, which can run a range of experiments that are normally done at a scale 50,000 times larger.
Welcome to the new frontier of digital cell biology, an emerging technology with immediate uses and abundant long-term potential. The chip and its related devices and software were invented by Berkeley Lights. The first company to embrace the new tool and apply it to biotech R&D was Amgen.
“I would say that it’s already a fairly big deal for us,” said Philip Tagari, Amgen’s VP for Therapeutic Discovery. “We’ve actually put this technology into practice in our antibody discovery work, and it takes about four months off the normal timeline. It’s also our standard method for cell line development, and we’re looking to extend our success in these areas to other applications. It’s starting to feel like a pretty transformative tool.”
In the near-to-medium term, digital cell biology offers scientists a faster and less resource-intensive way to run standard types of biotech experiments. In the long run, it has the potential to do for biology what sequencing did for genetics—make it possible to generate and analyze mountains of data to unravel the complexities of disease.
“I think we’re still very much at the tip of the iceberg in terms of what we can do with the technology and what people will discover by using it,” said Keith Breinlinger, chief technology officer for Berkeley Lights.
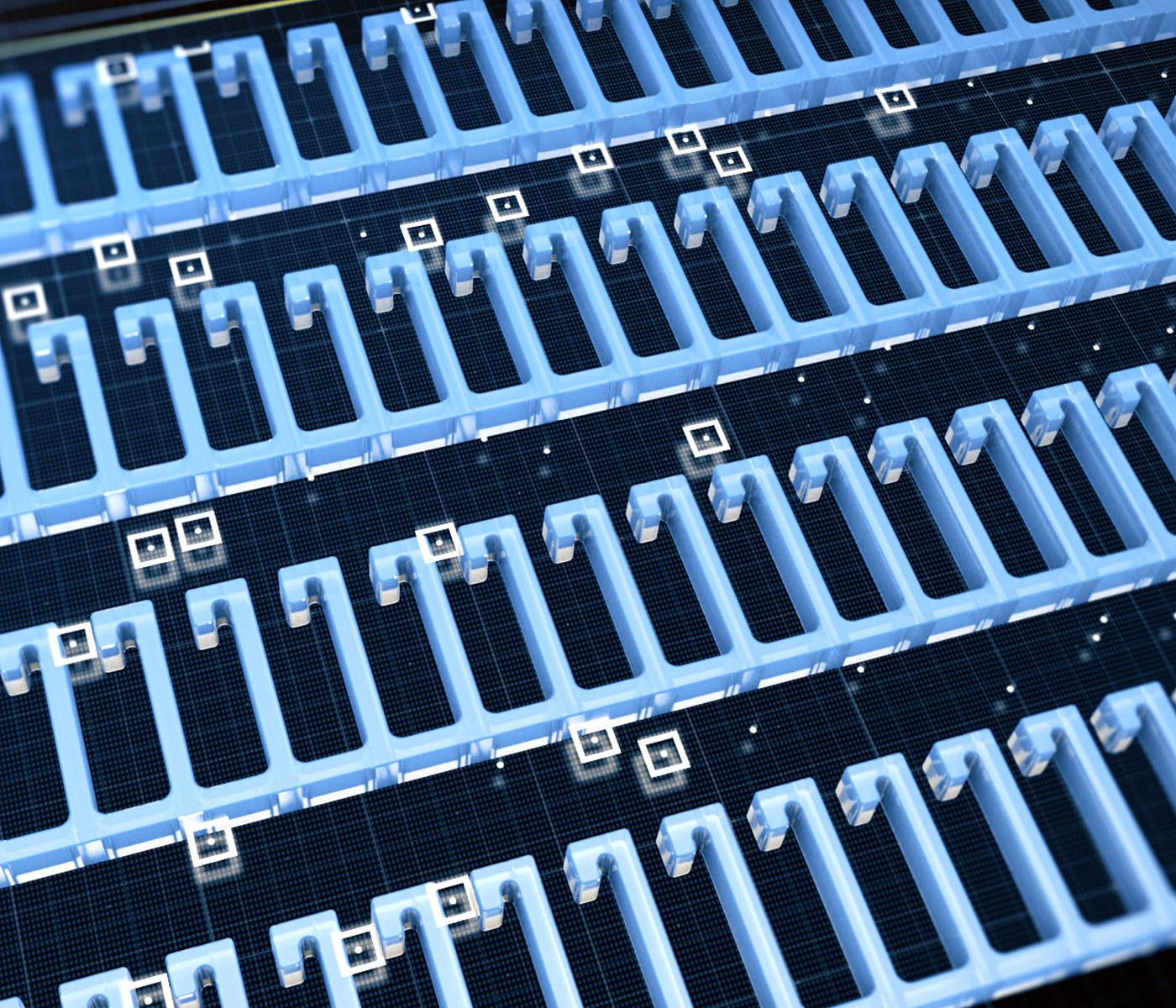
Cell-Friendly Optical Tweezers
The science behind the platform was inspired by a laser-based technology known as “optical tweezers” which earned Arthur Ashkin a share of the 2018 Nobel Prize. Optical tweezers can be used to precisely manipulate a single bead or cell. Ming Wu, one of Berkeley Lights’ founders, was a professor at UCLA when he started to develop a new and greatly improved optical manipulation technique.
Ming’s new optoelectronic tweezers require about 10,000 times less power to manipulate micron-scale objects, which makes the technique suitable for manipulating living cells without damaging them. Whereas optical tweezers use a lensing effect to direct photons and push a cell toward the center of the laser beam, optoelectronic tweezers use light to activate photosensitive switches that create local dielectric forces that repels cells. And unlike optical tweezers this manipulation can be performed on hundreds or thousands of cells in parallel. As the two techniques use different physics and to avoid further confusion, Ming’s technique was renamed “OEP” or optoelectronic positioning.
When Tagari visited Berkeley Lights at the invitation of chief science officer, Kevin Chapman, a former colleague, he saw the platform’s potential in biotech R&D right away. “At that time, they had four people in a portable building by the water in Emeryville, California,” Tagari recalls. “In that first visit, we sketched out what Amgen would want from the technology. About three months later, we signed a milestone-driven collaboration, and Amgen started to work very closely with Berkeley Lights and educate them on the details of antibody discovery.”
While companies have been making antibody-based drugs for decades, the standard discovery methods are far from ideal. For starters, the immune system’s antibody-producing B cells are “terminally differentiated.” That means they don’t proliferate or live very long, which has made them impractical for antibody production. Scientists get around this problem by fusing B cells to myeloma cells to create durable hybridomas. While this approach works, it has drawbacks.
“With hybridoma fusion, you’re jamming two cells into one and saying, ‘I hope your DNA plays nice together,’” says Aaron Winters, a scientist in Amgen’s Therapeutic Discovery group. “In the best-case scenario, only one out of every 1,000 B cells survives that process. So right off the bat, your efficiency in antibody discovery is 0.1 percent.”
Once you create stable hybridomas, it can take weeks to grow the millions of cells needed to start searching for drug-like antibodies. That search involves a “step-by-step and labor-intensive process,” said Marsela Jorgolli, a scientist in Amgen’s Hybrid Modality Engineering group. “There are some great tools and technologies available, but they are all highly dedicated to one particular aspect of antibody discovery or engineering.” Scientists spend a lot of time pipetting cells into flasks or plates, and ferrying plates between different devices designed to provide one piece of a larger puzzle.
Results In Days, Not Months
Jorgolli was part of a small team of scientists who served as a bridge between Amgen’s biologists and the Berkeley Lights engineers tasked with developing the platform’s capabilities. “We couldn’t just transfer our old procedures to the Berkeley Lights platform because the technologies are totally different,” she said. “We had to figure out how to tailor new procedures on the chip that would give us similar results, but much faster and more efficiently.”
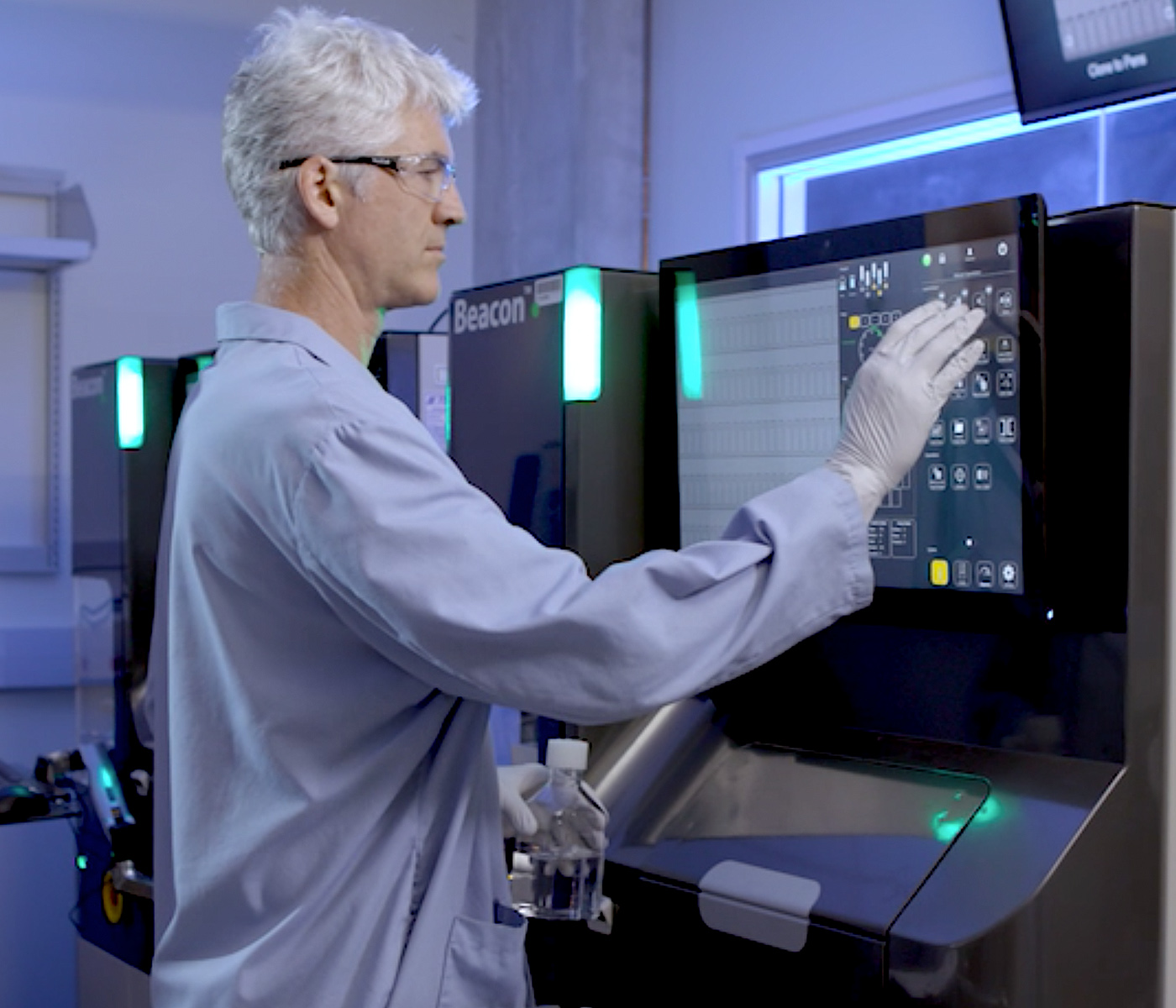
Aaron Winters running antibody discovery assays on the Beacon® platform.
As the collaboration progressed, Amgen received the first full-scale prototype device in 2013 and the first commercial device, known as the Beacon® platform, in 2016. “Just before we shipped that first Beacon to Amgen in December, the entire company signed it,” Breinlinger recalls. “All our signatures are on it under the hood.
Many benefits of the platform envisioned at the outset have come to fruition. For starters, the chips can miniaturize and integrate experiments that are normally done on several different devices. You can quickly see which cells are making antibodies, if the antibodies bind to their target, and if that binding has the desired impact on the target’s biology. “We are getting the data we need in hours or days, rather than weeks or months,” said Jorgolli.
In addition, the chips eliminate the need to create and grow large numbers of hybridoma cells. In the Beacon’s scaled-down NanoPens™, individual antibody-secreting B cells can thrive long enough to generate useful data. Scientists can then snip out the antibody genes from the best B cells and put them into more durable cells. The top-performers provide the cell line used later to support large-scale clinical testing and manufacturing.
“So That’s What’s Happening!”
The Berkeley Lights technology has become Amgen’s standard platform for cell line development, where it helps researchers to overcome the challenge of keeping cells happy. “Cells don’t like to be by themselves―they like to have friends around,” said Jennitte Stevens, a director in Amgen’s Process Development group. “They send out signals that help each other to grow. Dropping a cell into a 96-well plate is like dropping a person in an ocean. It takes weeks for them to get going again.” Cells prefer the cozier confines of NanoPens and grow better in that environment.
Standard cell line development methods are also labor intensive. Hundreds of plates are needed to increase the odds of finding a good cell line. Every well in every plate must be visually inspected to ensure it holds one cell and one cell only. With the new technology, “we don’t have to carry stacks and stacks of plates and put them in the imager and stare at them because the software does this for us,” says Stevens. “It also allows us to see how well the cells are growing and how much antibody they are producing at a very early stage of clone development. We can see things we weren’t able to capture before, like cells with really high levels of productivity and other unique attributes.”
The ability to visualize biology is one of the tool’s most attractive features. “Normally when you conduct an experiment, you can’t see what is really happening,” said Breinlinger. “You mix some things together, you get a result, then you try to infer what has happened, but you can’t visualize it. With our technology, you can actually see the cells and say, ‘So that’s what’s happening!’ You can solve a problem on day one instead of taking months to figure out why an experiment isn’t working.”
While the Berkeley Lights technology is now in numerous research organizations, Amgen and Berkeley Lights have maintained their close collaborative relationship. The goal is to keep expanding the range of experiments the platform can support.
“At our user group meetings, Amgen scientists will show us new assays they’re doing that the Berkeley Lights team didn’t show them how to do—they just figured it out on their own,” said Breinlinger. “They might ask us to tweak our software to help them do it more efficiently. We’re constantly working closely with our customers to push the envelope.”
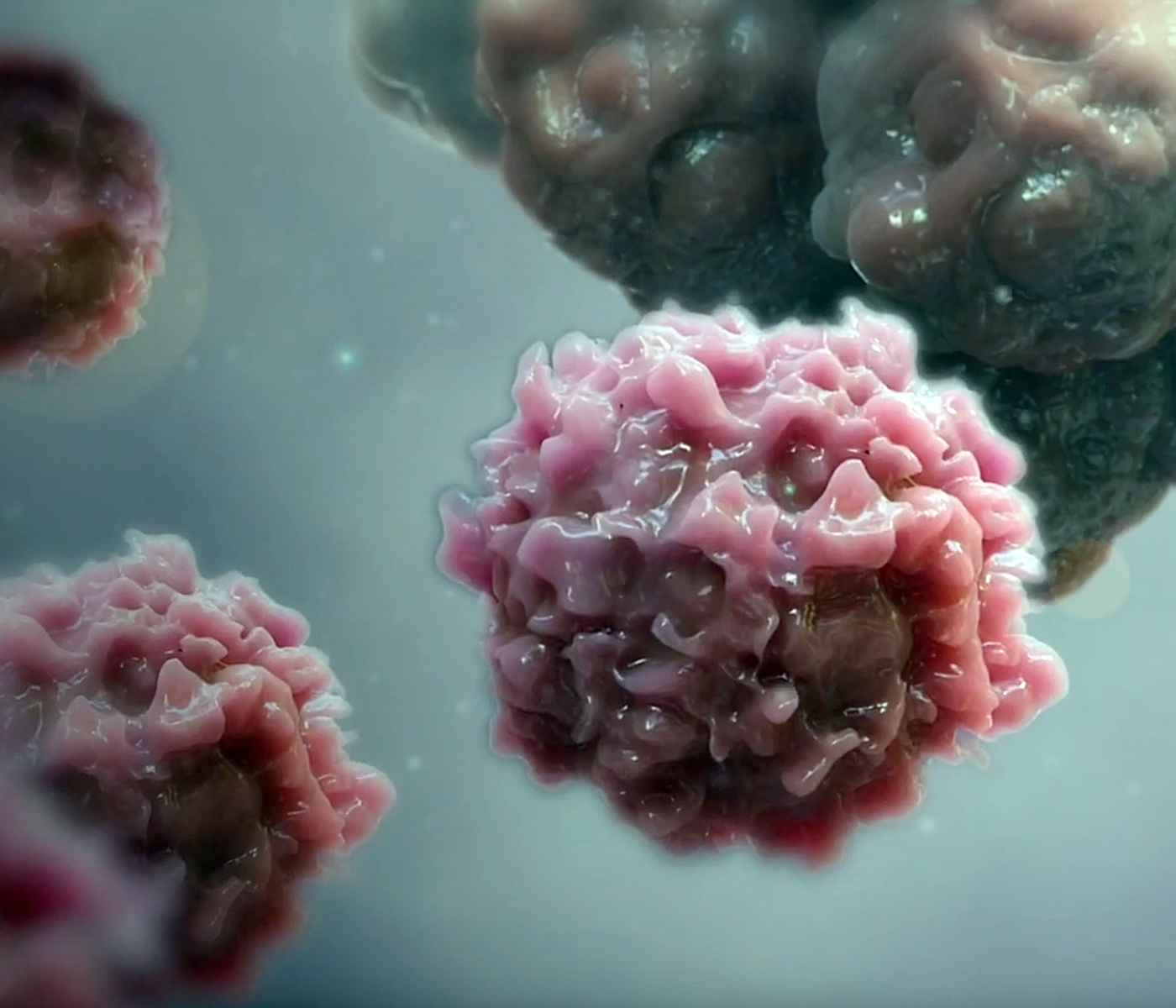
Single-cell experiments could yield new insights into the ability of individual T cells to fight cancer and guide the development of new immuno-oncology therapies.
Future Applications
The technology could be especially useful in immuno-oncology, where the goal is often to prompt T cells to attack tumor cells. “In immunology, we talk about T cell fitness,” said John Ferbas, a director in Medical Science at Amgen. “How many T cells do you have that are even capable of killing tumor cells? That’s a difficult question, but with this type of platform, we can do single cell assays to better understand T cell fitness in general and in individual patients. We can actually get video of what’s going on and learn a lot in a short time.”
The platform could also speed up research into rare subtypes of immune cells that are hard to isolate in large quantities. “If you’ve collected 20,000 rare immune cells, that’s only enough for about 20 experiments using standard microplates,” Tagari noted. “On the chips, you could do a thousand or more experiments driven by the software and get insights into the function of these cells far more quickly.”
In the long run, the platform could address a bottleneck in the effort to translate genetic insights into new therapies. Human genetics can tell us which genes and proteins have a major impact on disease risk, while biology is needed to clarify the function of newly discovered proteins and how best to target them. However, while DNA sequencing technology has become much faster and cheaper, we haven’t seen an equivalent productivity surge in biology. Discerning a protein’s function remains a slow and pain-staking process that can take years.
Digital cell biology may offer a solution to this problem, especially if the power of artificial intelligence can be applied, said Tagari. “In the future, I can imagine a day when computer-designed experiments can look at a thousand different variables in thousands of individual cells on chips. This technology will eventually allow us to do very complicated, quantitative cell biology at an unprecedented scale.”