1. Antibodies are Y-shaped proteins made in great abundance by our immune system.
While antibodies come in a few shapes and sizes, the most familiar of these are the Y-shaped proteins known as IgG antibodies (immunoglobulin G). The two upper arms of the Y have identical binding sites at their respective tips, and these sites are optimized to latch on to a specific foreign protein. The binding sites are also called the variable domains, because different antibodies have different tips to effectively target different threats. In the context of immunity, foreign proteins are collectively known as antigens.
The base of the Y is shared by all IgG antibodies. It’s called the Fc region because it binds the Fc receptors found on various immune cells in order to trigger a broader attack against the foreign threats recognized by antibodies. Once the immune system gears up to make antibodies, it makes a lot. Each of our immune B cells can secrete about 2,000 antibodies per second.
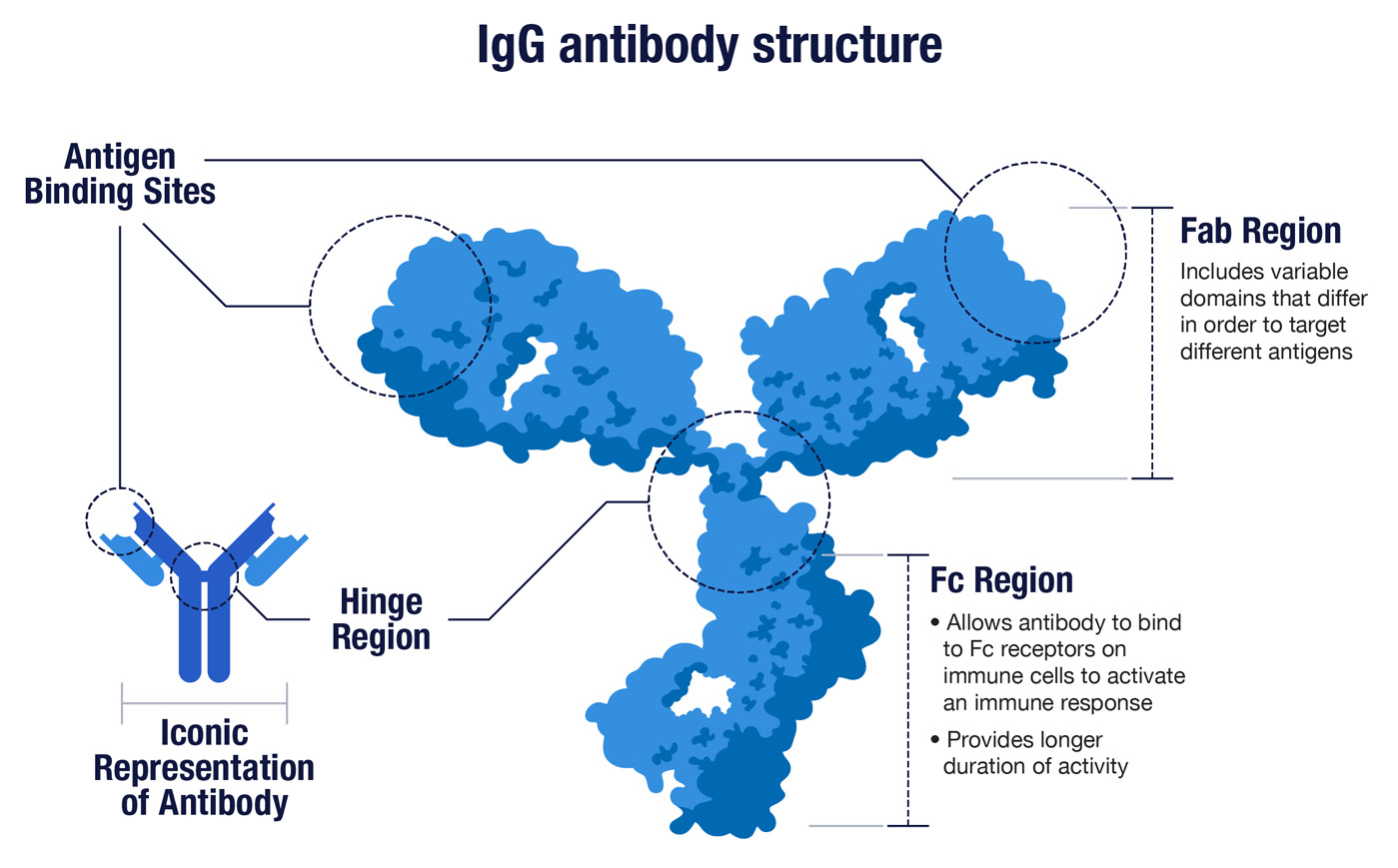
2. Antibodies work through a principle known as shape complementarity.
Just as a key only works if its shape conforms to the shape of the tumblers inside a lock, an antibody can only bind well to an antigen with a complementary shape. The real question is, How is our immune system able to make antibodies against the vast array of uniquely shaped foreign proteins found on the microbes we encounter in life? The answer is that we have trillions of lymphocytes, mainly B cells and T cells, and each equipped with its own uniquely shaped receptors.
“If these cells were all in one place, they’d form an organ about as big as the brain or the liver,” said Ray Deshaies, Amgen’s senior VP for Global Research. “But instead, they’re spread in different places like our bone marrow, spleen, and lymph nodes, so that they can rapidly detect and respond to foreign invaders to fight them off.” When these receptors encounter a foreign antigen they can bind to, the binding event triggers an immune response that eventually produces antibodies.
“With SARS-CoV-2 for example, your immune system doesn’t ‘know’ in advance that it will be infected,” said Deshaies. “So instead, it tries to cover all the bases by making as many different B and T cells receptors as possible. It’s a bit like ensuring you win the state lottery by purchasing tickets for all the possible number combinations. If that sounds incredible, it’s for a good reason—it is!”
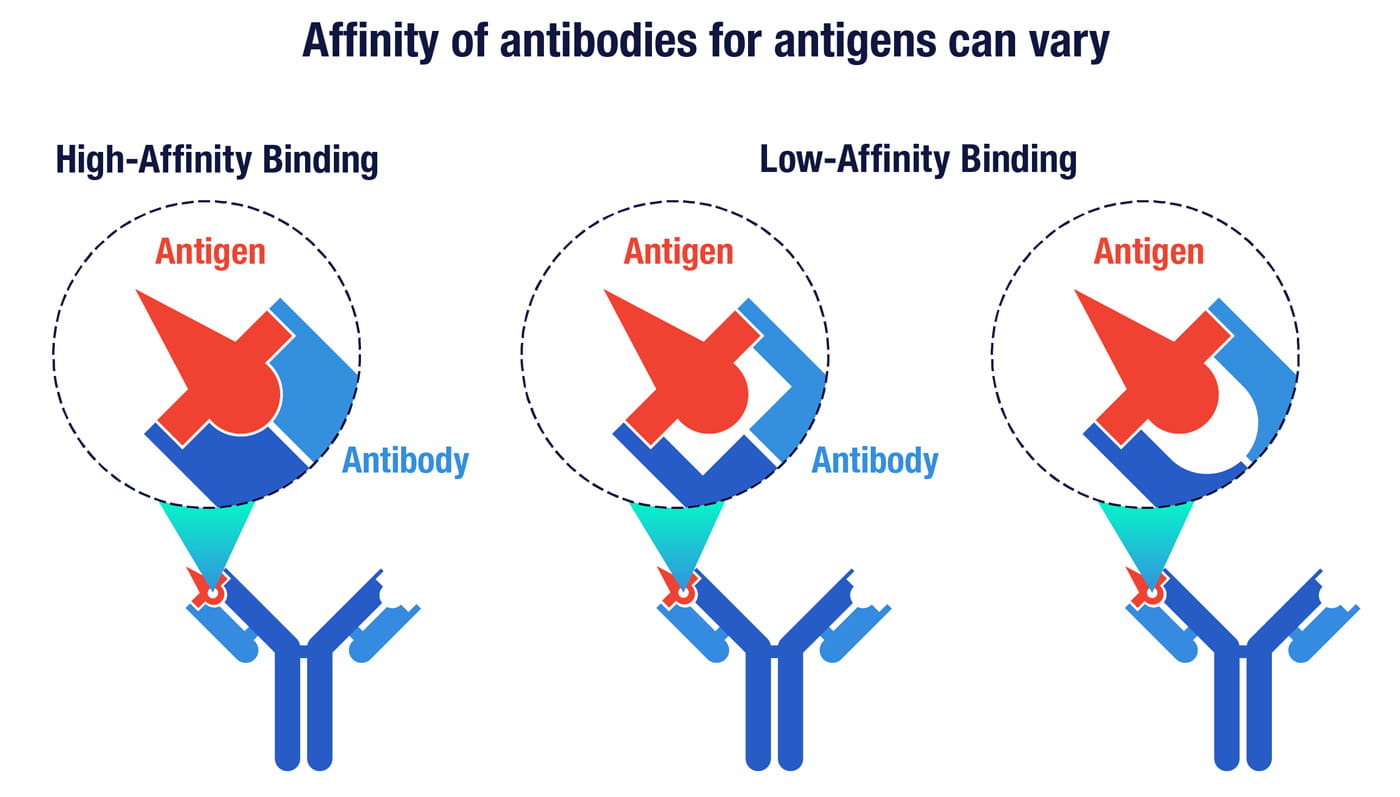
The affinity of an antibody is a measure of its surface complementarity with a target antigen. Antibodies with higher affinity have a higher degree of shape complementarity that enables them to bind more tightly to their targets.
3. Antibodies are slow to develop but offer long-term protection.
Our bodies have two layers of immune protection—innate immunity and adaptive immunity. You see the innate response in the redness and swelling around a wound, as distress signals from infected cells cause blood vessels to dilate and grow leakier to help immune reinforcements reach the wound. This initial but relatively non-specific response buys time for adaptive immunity to mount a potent and targeted counterattack.
That attack begins when dendritic cells—the vacuum cleaners of innate immunity—collect and chop up bits of foreign protein they encounter. “The dendritic cells then scamper away to the nearest lymph node and present bits of foreign proteins on their surface to T helper cells,” said Deshaies. “It’s as if they’re saying, ‘Hey, look what I found!’ And because there are billions to trillions of different T helper cells, chances are one of them will have receptors that can bind the antigens that get displayed.”
Adaptive immunity is so strong, we have a two-stage fail-safe mechanism to ensure it’s only aimed at true threats. To trigger the adaptive response, a T helper cell and a B cell must each encounter and bind to the same foreign antigen. Only then will the T helper cell signal its B cell partner to go on the offensive. The unleashed B cell starts dividing into numerous clones of itself. Some clones turn into antibody-secreting factories known as plasma cells, while others mature into long-lasting memory B cells. The antibody response can take a couple of weeks or longer to reach optimal potency, but memory B cells stay in the body to ensure a rapid counterattack if the same threat appears again.
4. B cells have a mechanism for boosting the binding power of antibodies.
Why does it take time to generate optimal antibodies to threats like coronavirus? The answer, in part, is that B cells don’t want to settle for just any antibody—they want the very best antibodies they can muster. The challenge is that even though there are up to trillions of different B cells, there are many more possible foreign protein shapes to guard against. So when a B cell becomes activated, the fit for its targeted antigen is likely to be good but not perfect.
However, when that B cell starts dividing to create clones of itself, the genes in these cloned cells are sprinkled with mutations that produce antibodies that are highly similar but slightly different from the original parent cell. “As they proliferate with T-cell help, the B cells can make millions of different flavors of the same antibody receptor,” said Deshaies. “Many of these new receptors will bind the original antigen but with different affinities. The one that binds best will proliferate the most because it gets activated the most.”
This process, called affinity maturation, can ratchet up the binding strength of antibodies by more than a thousand-fold. This tight fit is one hallmark of a great antibody, but it’s not the only success factor.
When an activated B cell starts dividing, many of the progeny cells will have antibodies with similar affinity or less affinity than the original parent cell. But a few will have antibodies with much higher affinity, and the cells that bind best to the target will proliferate.
5. Not all antibodies are created equal.
An antibody’s effectiveness against viruses and other threats depends not only on how strongly it binds but where it binds. “It’s not like our immune system is intelligent and knows beforehand exactly what antibodies to make,” Deshaies observes. “It just makes any possible antibodies it can against any viral antigen it can see.”
The result can be thousands of different antibodies that bind to different proteins on a virus or different parts of the same protein. Some of these antibodies might not deter the virus at all, while others may block key proteins, for example the spike protein the coronavirus uses to infect cells. Antibodies that defend cells from infection are called neutralizing antibodies.
Even antibodies that don’t block infections directly have their value, said Deshaies. “If something is coated with antibodies, your innate immune system will assume it’s probably foreign. There are cells in the innate immune system called macrophages, which vacuum up and digest things that are coated with antibodies in a process known as phagocytosis, which literally means cell eating.”
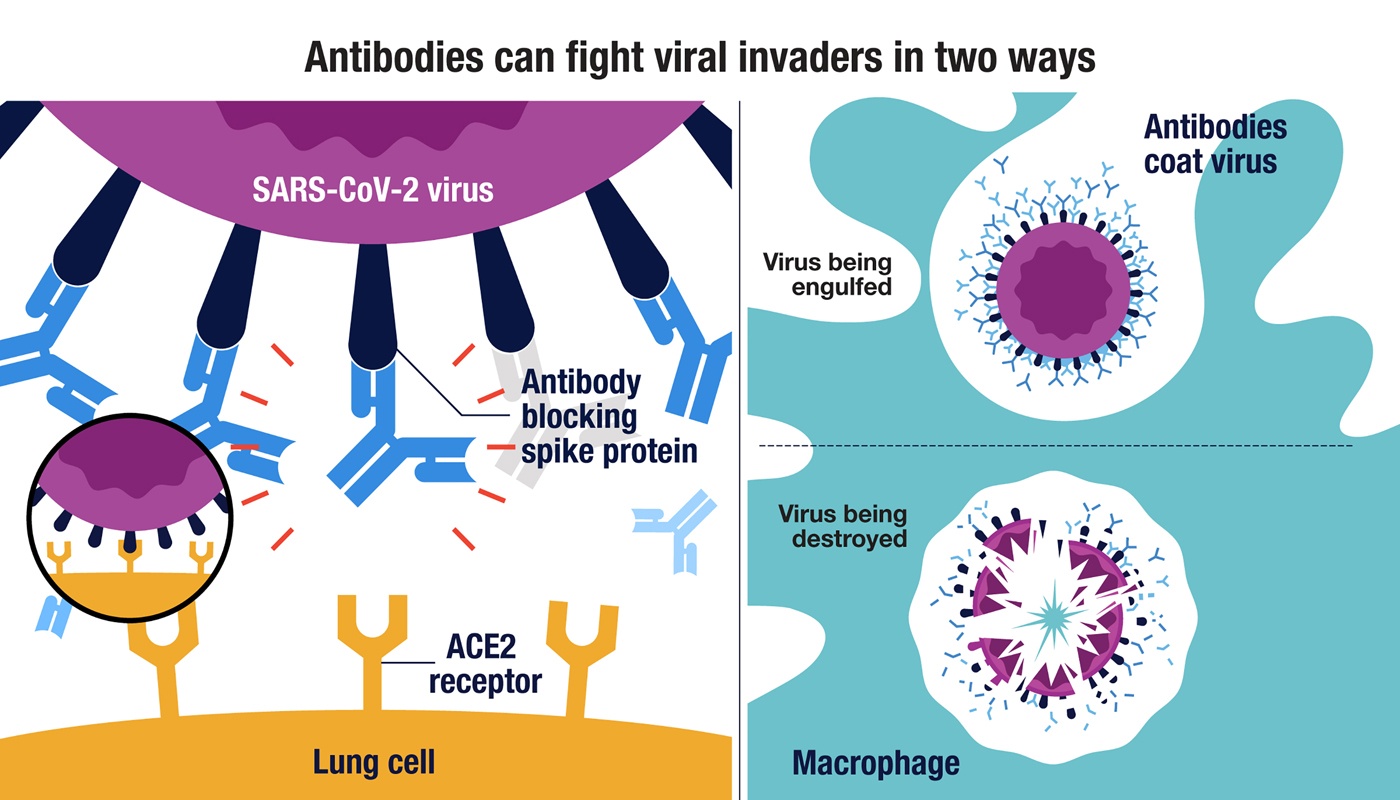
(Left side) The SARS-CoV-2 virus can enter lung cells when the virus’s spike protein bind to the ACE2 receptor on the cell surface (insert). Antibodies that block the spike protein can interfere with this binding and neutralize the virus. Antibodies that bind to other SARS-CoV-2 proteins can also help to fight infections by signaling macrophages to engulf and destroy the virus (right side).
6. There are two ways to accelerate the protection provided by antibodies.
In some people with a viral infection, the antibody response may arise too weakly or too late to fight off the virus. In other cases, the antibodies made by the immune system may bind tightly to the virus but fail to neutralize it. There are two main therapeutic approaches that can augment or strengthen a person’s natural antibody response.
A vaccine, based on inactivated virus or viral antigens, can prompt your immune system to recognize a virus and produce memory B cells against the virus to guard against a future infection. Vaccines potentially offer long-lasting active immunity, but it may take weeks or months for that protection to develop.
Neutralizing antibodies that target a virus can also be manufactured outside the body and injected into patients or those at high risk of infection to potentially treat or prevent a disease. Therapeutic antibodies can offer rapid protection, but this passive immunity may only last for a few weeks to a month before the injected antibodies are removed by natural processes. (The antibodies your body makes are also removed eventually, but your immune system can make more as needed.)
While vaccines may offer the best long-term solution to viral infections, other therapies may still be needed even after vaccines become widely available. This is because vaccines may not provide lifelong immunity, or they may be only partially effective in some patients. In instances where immunity wanes or never fully develops, an antibody therapy could prove useful.
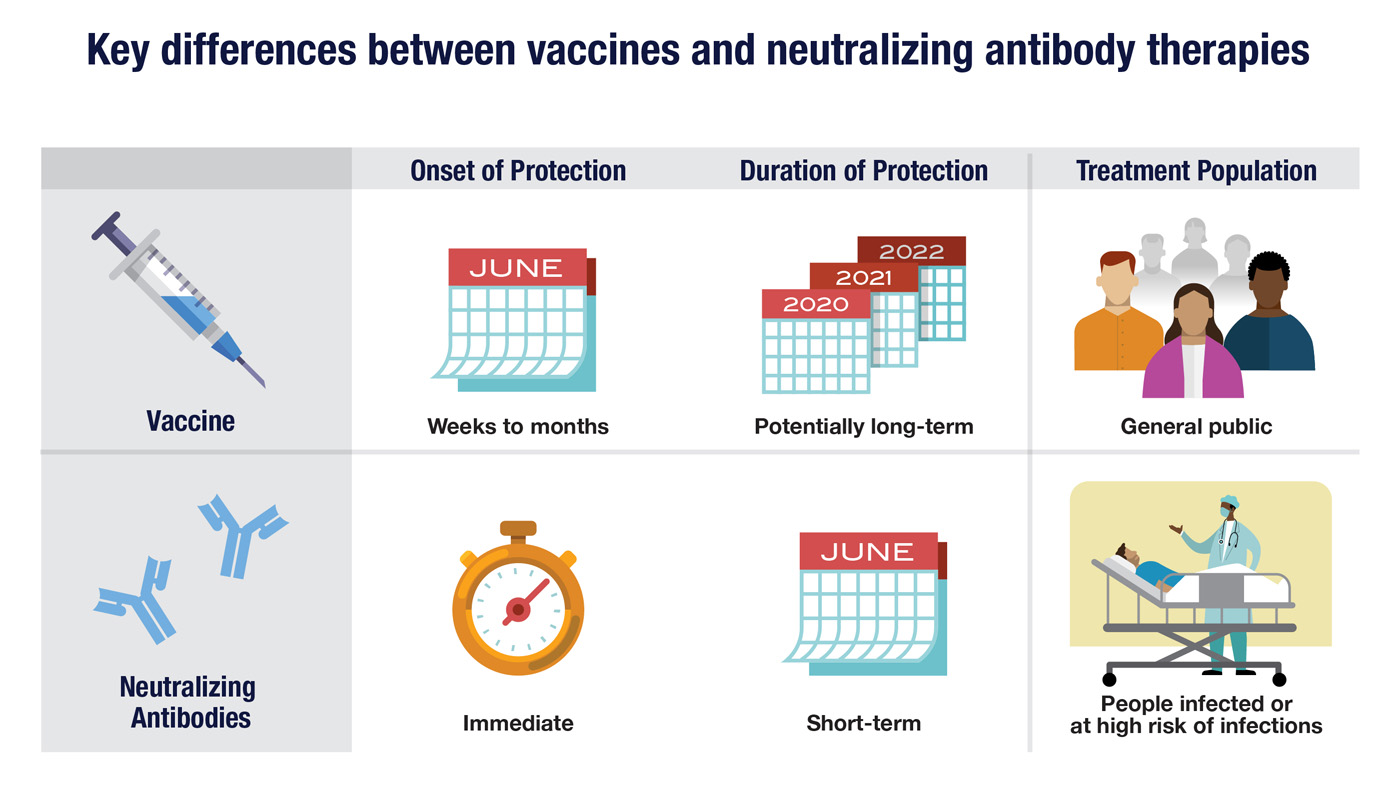
7. The approaches for providing passive immunity are evolving.
There are several ways that antibodies made inside one person can be used to treat viral infections in other people. The oldest and simplest approach is to collect blood plasma from donors who have recovered from an infection and give it to people infected with the same virus. While this approach may help at least some patients, it has drawbacks. Convalescent plasma can vary significantly in its potency and quality, and plasma from one recovered patient can only be used to treat a few people at most.
Neutralizing antibodies can be made at larger scale through the same techniques used to produce other types of antibody-based therapies. The steps include isolating and purifying the target antigen, injecting that antigen into mice with humanized immune systems, and then testing the antibodies the mice produce to find those that bind with high affinity to the target. The genes that code for these high-affinity antibodies can be inserted into cell lines designed to function as antibody factories.
Finally, you can use antibody genes taken directly from individuals who have had an effective response to a virus. By isolating and testing plasma cells or memory B cells from these people, it may be possible to find genes that produce highly potent neutralizing antibodies. This approach may take more work upfront, but it could deliver results worth waiting for.
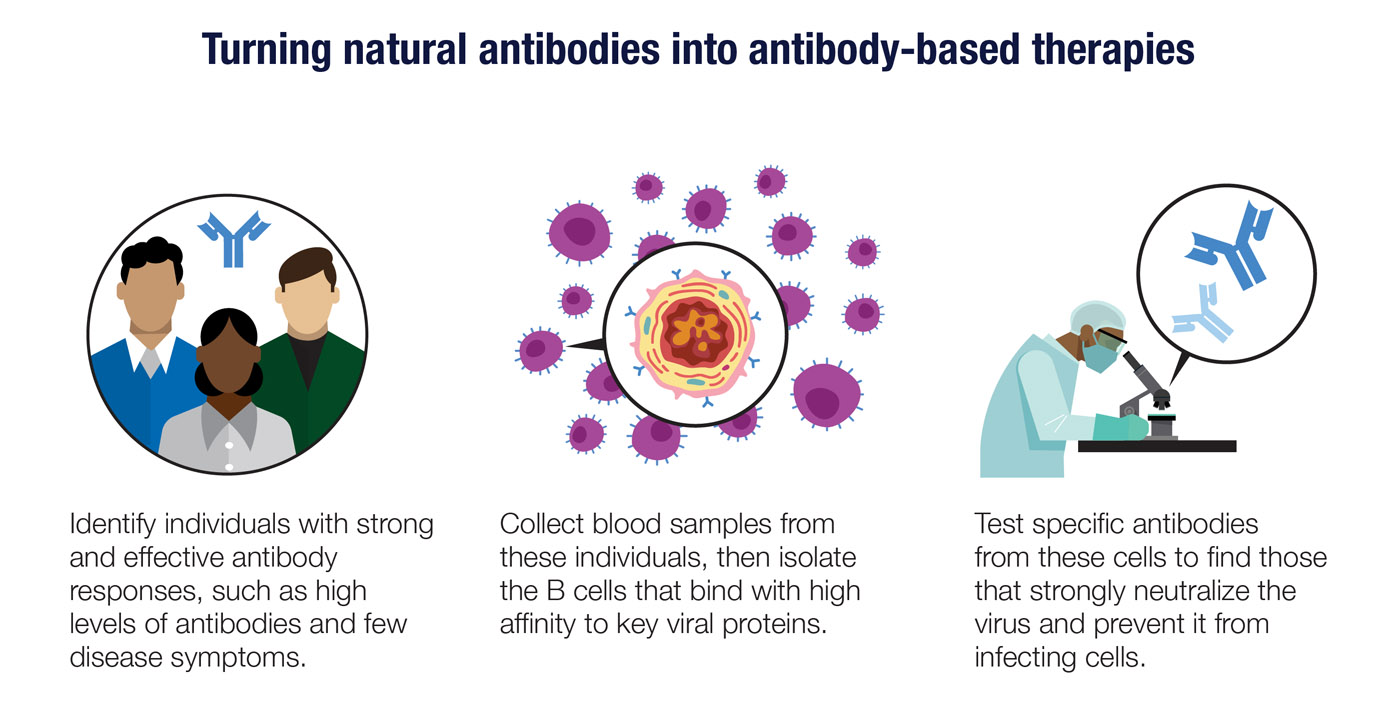
8. Viruses are often moving targets for a vaccine or an antibody.
One challenge in targeting any virus is that they don’t stand still—they mutate. For example, in sequencing the genomes of viral samples obtained from Icelanders infected with SARS-CoV-2, Amgen’s deCODE Genetics subsidiary found 409 mutations, including 291 not reported previously.
Since antibodies require shape complementarity to work, mutations that change the shape of viral proteins can limit an antibody’s effectiveness. In designing a neutralizing antibody, it’s important to have up-to-date knowledge of how the virus is changing. You want to make sure you are targeting viral proteins or segments of proteins that are less likely to have mutations. Cocktails of several different antibodies may be needed to cover the majority of viral strains that have evolved around the world.
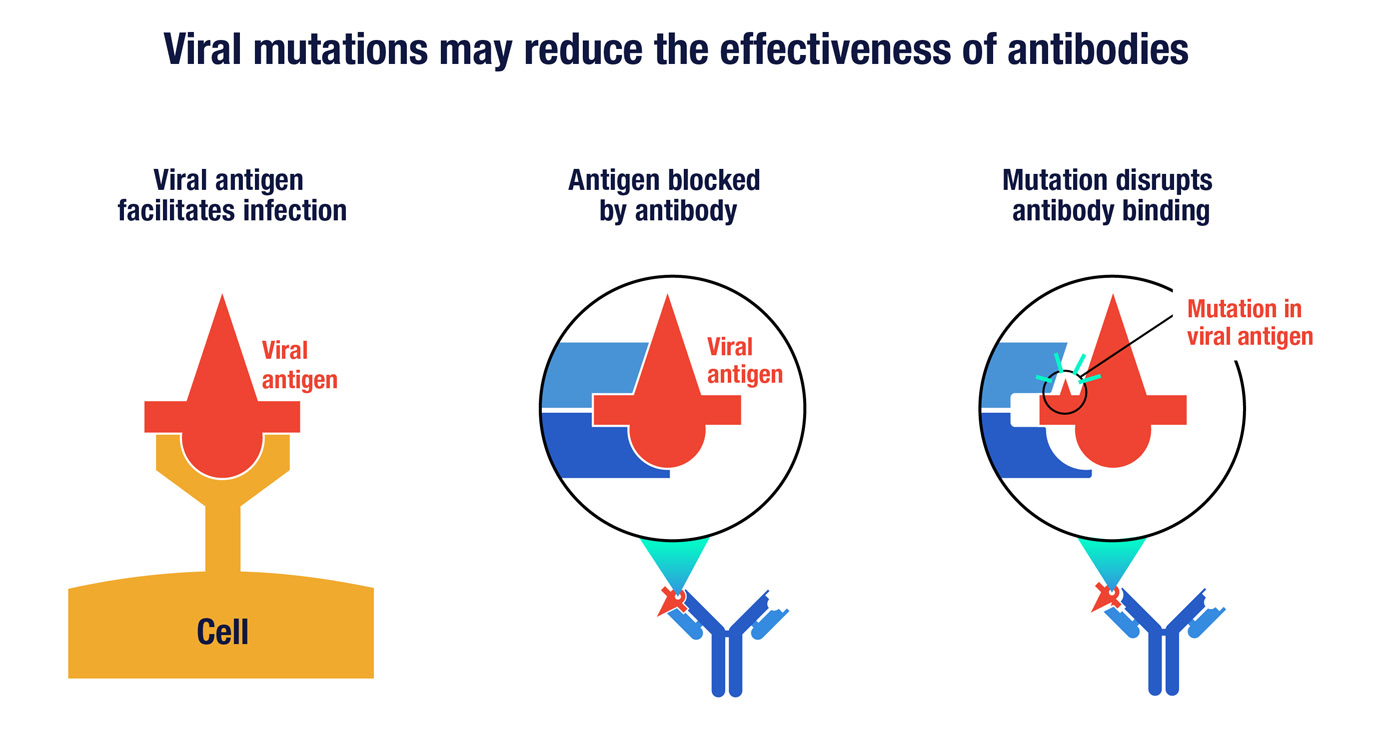
A key viral antigen, represented here by the symbol in red, can enable the virus to infect human cells by binding to a particular receptor (left). A neutralizing antibody can prevent infection by binding to the viral antigen in a way that disrupts its ability to engage the cell receptor (middle). However, random mutations in the antigen can potential thwart antibody binding (right) without altering the virus’s ability to infect cells.
9. Antibodies can be engineered to enhance their performance as medicines.
Just as B cells have evolved a way to ratchet up the quality of their antibodies, biotech scientists have also developed an antibody-enhancement toolkit. Once an antibody that binds to a target antigen is identified, molecular engineers can apply lessons learned from decades of antibody design and development..
An antibody’s attributes depend on its precise three-dimensional structure, which in turn depends on the sequence of DNA letters in the antibody gene. Scientists can tweak the structure by modifying the genes, giving rise, for example, to an antibody that is easier to manufacture. Other changes can lead to antibodies that stay longer in the body or have higher affinity for their target antigens. Changes to the antibody’s Fc region—the base of the Y-shaped molecule—help to determine the antibody’s distribution in the body and its ability to activate innate immune cells like macrophages.
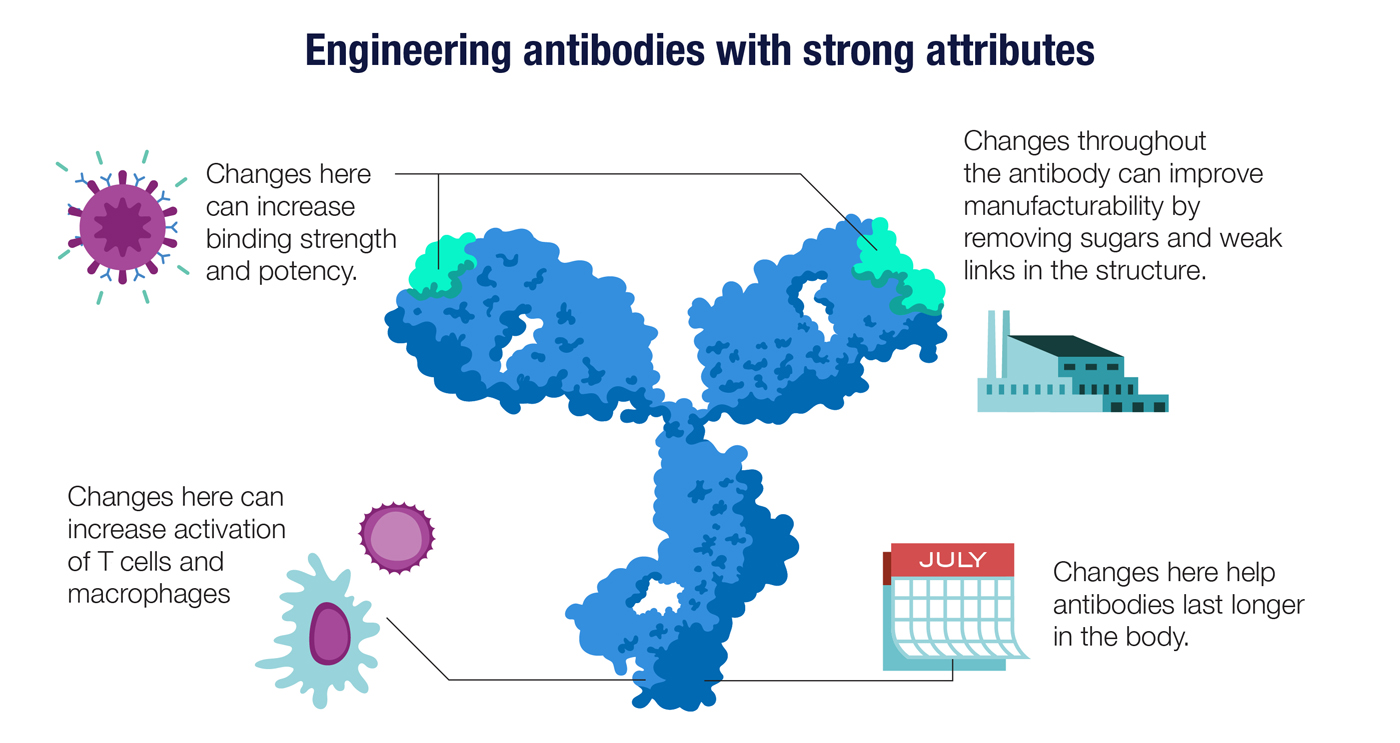
10. There have been major improvements in antibody manufacturing.
Manufacturing antibodies is a science in its own right, and it starts with transforming cells that didn’t evolve for this role into antibody factories. Given their size and complexity, antibodies can only be made by cellular machinery, and the cell lines that work especially well are derived from ovaries of Chinese hamsters. These CHO cells are genetically engineered to produce fully human antibodies, and to do so with a relentless intensity similar to our own B cells.
Amgen has been at the forefront of advances in biomanufacturing, developing more productive cell lines with high antibody yields as well as processes that keep these cells healthy and productive at higher densities. These improvements and others have allowed us to redesign biotech manufacturing to make it leaner and greener as well as more flexible and productive.
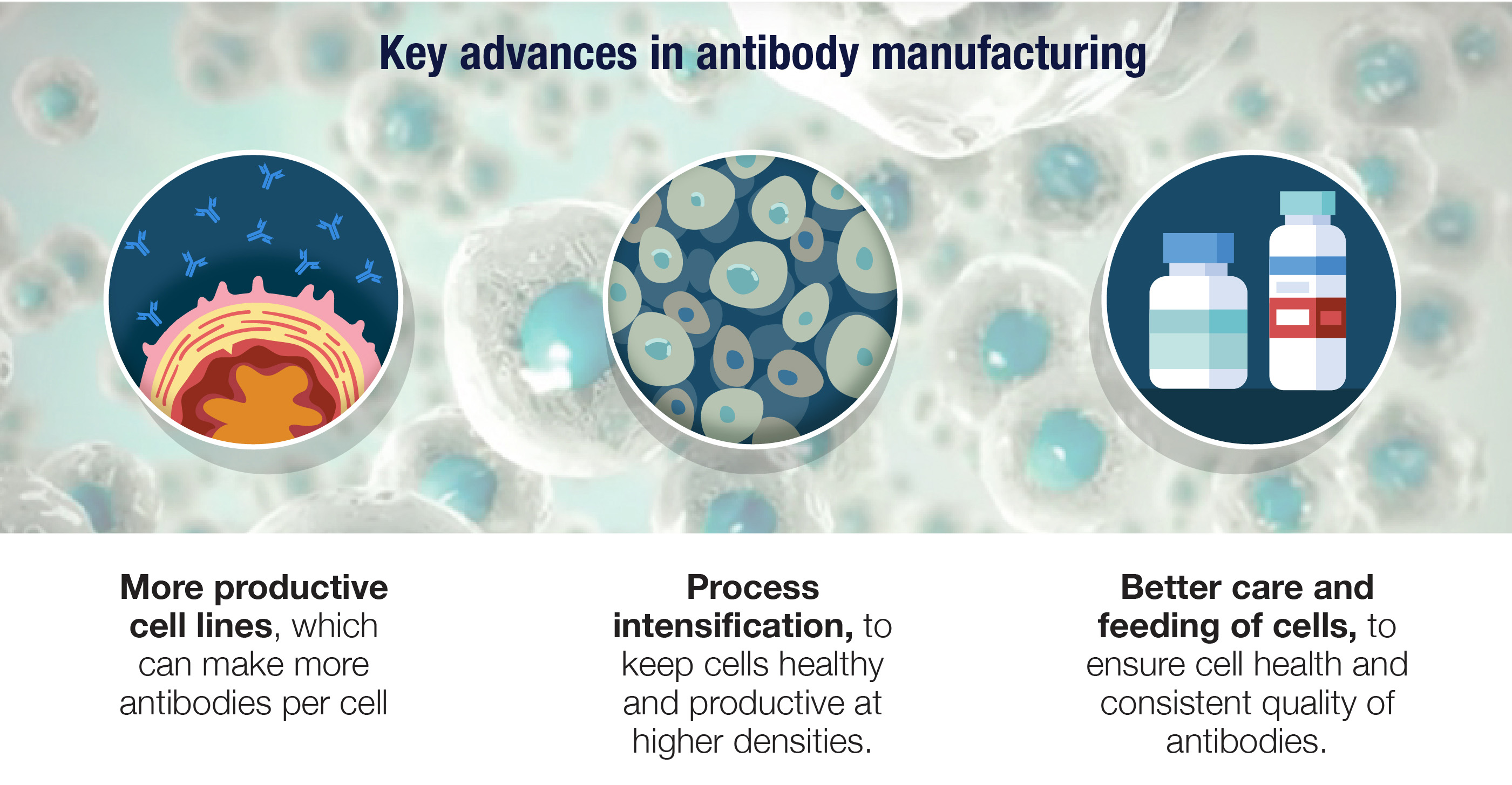